- Home
- Classes
- Physics 134 – Observational Astrophysics – Fall 2022
- Physics 6B – Spring 2015
- Physics 134 – Observational Astrophysics – Fall 2020
- Symmetry & Aesthetics in Contemporary Physics
- Physics 128 – Senior Lab – Fall 2016
- INT 184 – PL Interdisciplinary Honors Seminar – Weapons of Mass Destruction – Spring 2013
- Physics 150 – Modern Design and Fab – Fall 2019
- Physics 134 – Observational Astrophysics – Fall 2019
- Physics 2 – Fall 2013
- Physics 3 – Fall 2016
- Physics 4 – Winter 2020
- Astro 1 – Fall 2020
- Physics 4 – Winter 2023
- Physics 141 – Optics – Spring 2019
- Physics 145L – Astrophysics Research
- Physics 199 – Independent Research
- Projects
- Facilities
- People
- Net
- Outreach
- Misc
- Papers
PI – Multimodal Planetary Defense
Links to papers:
Detailed technical paper:
PI-Terminal-Defense
arXiv archive link:
http://arxiv.org/abs/2110.07559
ASR – Advances in Space Research – 10-22
https://www.sciencedirect.com/science/article/pii/S0273117722009395
Scientific American Article:
https://www.scientificamerican.com/article/planetary-defense-is-good-but-is-planetary-offense-better
UCSB Press Release 10-11-21:
https://www.news.ucsb.edu/2021/020432/planetary-defense-pi-sky
Summary Briefing Charts:
PI – Lubin-l
Don’t Forget to Look Up 1-25-22
A Physicist’s View of Hollywood and Existential Threats
https://arxiv.org/abs/2201.10663
CBS Coverage on the “Don’t Forget to Look Up” paper (many others on the net with less fidelity)
https://www.cbsnews.com/news/asteroid-dont-look-up-nuclear-explosives/
UCSB Deepspace YouTube Channel – PI Movies and Additional Materials
www.youtube.com/@UCSBDeepspace
UN Meeting on Planetary Defense – PDC 2023 – Vienna -April 2023
https://www.unoosa.org/oosa/en/ourwork/topics/neos/2023/IAAPDC/index.html
PI talk at the end of session 8 April 6, 2023
https://media.un.org/en/asset/k11/k112i49pj8
List of Acoustic and Optical Ground Effect Simulations on Youtube
List-of-Acoustic-and-Optical-Simulations
List of Hypervelocity Intercept Simulations on Youtube
List of Hypervelocity Intercept Simulations
π
Multimodal Planetary Defense
PI is a practical and effective method of planetary defense that allows for extremely short mitigation time scales if needed as well as general use for longer warning times cases. The method involves an array of small hypervelocity kinetic penetrators that pulverize and disassemble an asteroid or small comet. This effectively mitigates the threat using the Earth’s atmosphere to dissipate the fragment cloud where the atmosphere acts as a “bullet proof vest” or “body armor” for short warning time threats while the fragments miss the Earth for longer warning time threats. The effects of doing so are remarkable in their effectiveness. In the terminal defense mode the key is to de-correlate the resulting acoustic blast waves on the ground and to take into account the optical signature due to fragment “burnup”. Understanding both effects and and spreading the fragment cloud both spatially and temporally allows for virtually any threat to be mitigated. PI stands for “Pulverize It”.
The system allows a terminal defense solution to planetary defense using existing technologies. This approach will work in extended time scale interdiction modes where there is a large warning time, as well as in short interdiction time scenarios with intercepts of hours to days before impact. In longer time intercept scenarios, the disassembled asteroid fragments largely miss the Earth. In short intercept scenarios, the asteroid fragments of maximum ~10-meter diameter allow the Earth’s atmosphere to act as a “beam dump” where the fragments either burn up in the atmosphere or air burst, with the primary channel of energy going into spatially and temporally de-correlated shock waves. Compared to other threat reduction scenarios, this approach represents an extremely cost effective, testable, and deployable approach with a logical roadmap of development and testing. Pre-deployment of the system into orbit or a lunar base allows for rapid response on the order of less than a day if needed. The effectiveness of the approach depends on the time to intercept and size of the asteroid, but allows for effective defense against asteroids in the multi-hundred-meter diameter class and could virtually eliminate the threat of mass destruction caused by these threats. The great advantage of this approach is that it allows for terminal defense in the event of short warning times and target distance mitigation where orbital deflection is not feasible.
As an example, we show that with only a 1m/s internal disruption, a 5 hours prior to impact intercept of a 50m diameter asteroid (~10Mt yield, similar to Tunguska), a 1 day prior to impact intercept of 100m diameter asteroid (~100Mt yield), or a 10 day prior to impact intercept of Apophis (~370m diameter, ~ 4 Gt yield) would mitigate these threats. Mitigation of a 1km diameter threat with a 60-day intercept is also viable. We also show that a 20m diameter asteroid (~0.5Mt, similar to Chelyabinsk) can be mitigated with a 100 second prior to impact intercept with a 10m/s disruption and 1000 second prior to impact with a 1m/s disruption. Zero-time intercept of 20m class objects are possible due to atmospheric dispersion effects. The theoretical details and the analytic and numerical simulations showing the program works is discussed in our technical papers.
Summary of PI Mitigation Modes
1) Short time warning – minutes to days intercept – terminal defense (15m to 100m diam threats)
-
Fragment to <15m and use Earth’s atmosphere as body armor – shock waves de-correlated
-
Sum of all optical pulses below combustion limit – no fires
-
Shock waves de-correlated – virtually no damage – possibly some minor window damage
-
Ex: 100m diam (~100 Mt >> Tunguska) can be mitigated with 1 day intercept
-
Ex: 20m (0.5 Mt – Chelyabinsk) can be mitigated with 100 sec intercept (10m/s disruption)
2) Moderate time warning – 10-60 day intercept (100 – 500m – Apophis, Bennu)
-
Fragment to <15m and spread fragment cloud over large area on Earth (~ 1000 km radius)
-
Earth’s atmosphere is used as body armor
-
Ex: 350m diam (~Apophis) (~ 4 Gt ½ Earth nuclear arsenal) can be mitigated with 10 day intercept
-
Ex: 500m diam (~Bennu) (~ 8 Gt > Earth nuclear arsenal) can be mitigated with 20 day intercept
3) Longer time warning (>75 day intercept) (600-1000m threats)
-
Fragment ideally to <15m but less restrictive
-
Fragment cloud spreads to be larger than the Earth – Virtually all fragments miss the Earth
-
Residual fragments that will hit the Earth smaller than 15m are not a threat – atmosphere mitigates
-
Residual fragments > 15m can be dealt with as in option 1) terminal defense IF needed
4) Long term warning and existential threat (>100 day intercept and >1& <15km diameter)
-
Fragment using NED penetrator array – pure fission (eg W82 class NED) looks feasible – based on nuclear artillery technology already designed, developed and tested. Sequential penetrator option allows better NED effectiveness and possibly thermonuclear class penetrators if These are internal and NOT standoff detonations.
-
Possible use of “sequential following penetrators” to allow “hole drilling” for better NED coupling and lower “g” forces for devices such as B61-11 NED physics package – 4 kt/kg @ 350 Kt yield
-
Fragment cloud spreads large enough to miss Earth for virtually all fragments.
5) Long term warning (> 1 year) – asymmetrical fragmentation/ enhanced deflection option
-
Asymmetrical fragmentation to get extreme deflection enhancement – use energy not momentum.
-
Blast off part of target to use it (“push”) against itself – NOT like current deflection techniques but synergistic. See mode 6 below.
-
Depending on target size can use kinetic only, kinetic with conventional explosives or NED penetrators for extreme threats.
-
Use of penetrator to drive mass ejection via an induced “rocket exhaust” mode where the high temperature and pressure vaporized/ plasma material created inside the bolide exits through the penetrator initiated “exhaust nozzle” to form a rocket engine using the bolide as “fuel”.
6) Long term warning (> 1 year) – classical deflection
-
If desired the same system can also be used as a classical deflector -complete multimodal use.
-
Simple penetrator reconfiguration allows the same system to be used as a classical deflector.
-
In general this mode is not needed as mode 5 (enhanced deflection) is superior but it is available.
PI allows one system to be used from terminal defense to classical deflection modes
Such a capability would allow humanity for the first time to take control over its destiny relative to asteroid and comet impacts.
How does PI work?
PI works by intercepting the parent threat, whether asteroid or comet, by intercepting the parent bolide with an array of penetrating rods, some filled with conventional explosives, early enough to both fracture the parent bolide into small fragments that are typically below 15m diameter. The penetrators fracture and spread the fragments laterally as well as along the velocity vector direction. Only small fragment speeds (in the bolide frame) and hence low energy are required. The typically speed of the fragments in the bolide frame is roughly 1 -10 m/s. For reference you can walk faster than 1 m/s. Faster fragments speeds are better but require more energy. The resulting fragments are spread out into a fragment cloud that then eventually either hits the Earth’s atmosphere or if intercepted early enough the fragments miss the Earth completely. The fragment cloud enters the Earth’s atmosphere at hypersonic speeds (typically around Mach 60 or 60 times the speed of sound) and like a re-entering spacecraft it experience extreme levels of “ram pressure” and heating due to high speed impacts from the atmosphere, causing breakup due to structural failure of each fragment at altitudes depending on the fragment size (typically around 30-50 km altitude for our system). The resulting cascade effect causes a “detonation” with a large acoustical blast wave and optical pulse generated. The acoustical blast is similar to the “sonic boom” from a re-entering spacecraft or supersonic spacecraft. While the energy of each fragment can be similar to a modern strategic thermonuclear weapon there is no radiation hazard, only a large “light and sound” show. What could have killed millions had no intercept taken place is now reduced to a large number of displays of thunder and lighting like effects. The key is that the acoustic shock waves are de-correlated in time so an observer “hears” a series of small blast wave that rise and fall rapidly on a time scale of order a second and hence what would have been a deadly high pressure blast wave is reduced to a series of much lower pressure blast waves that do not overlap in time in general. There is also a small amount of dust generated from the fragment detonation in the atmosphere. We show that the dust is not significant in terms of causing a “nuclear winter” scenario.
Other Penetrator Options
There are many possible ways to accomplish the fragmentation/ pulverizing of the threatening bolide. Optimization of the various techniques we are considering will come from detailed hypervelocity simulations and later from ground testing. Methods we are considering, in addition to the longer penetrator rod, include large numbers of small explosive filled rods/ spheres that hit in waves to “blow off successive surface layers” as well as simple solid spheres (like metal “ball bearing” ) that impact and erode the bolide. The optimum techniques will also depend on the size and composition of the bolide. Multiple options could be “at the ready” with a simple swapping of the intercept payload depending on the threat. Ideally we will find a single option that works well in all cases.
Energy Required for Internal Disruption
As shown in our technical paper and in the briefing charts, the energy required for overcoming the gravitational (self attraction) energy of the bolide and the kinetic energy to propel the fragments into the spreading fragment cloud is very small. For example, a large 100m diameter rocky asteroid approaching the Earth at 20 km/s, only requires 250 kg of explosives (TNT equivalent) or a 5 kg mass of penetrator to supply the energy required to overcome the gravitational assembly energy and to spread the fragment cloud at a speed of 1 m/s. The reason that the penetrator mass is so small is that in the frame of reference of the bolide the penetrator has a speed of the approach of the speed of the bolide (20 km/s in this case) and thus the kinetic energy in the penetrator is extremely high, much higher than the equivalent energy of the same mass of chemical explosives. The critical question to be answered in our upcoming hypervelocity simulations is the coupling between the penetrator kinetic energy (in the frame of the bolide) and the kinetic energy of the fragments (in the frame of the bolide). While explosive filled penetrators add little in terms of the total energy in the bolide frame, they do offer the potential for much higher energy coupling into the bolide. More is needed in the simulations to determine if explosive filled penetrators are needed.
Energy Scale Comparison
Comparing energy scale is helpful in understand the basic problem and solution. Consider that the 2013 Chelyabinsk asteroid impacts with a diameter of approximately 20m had the energy of about 40 times that of the Hiroshima nuclear weapon. We know the blast wave and optical pulse measured in Chelyabinsk is fairly consistent with our calculations as discussed in the PI technical paper.
In PI, by spreading the fragments out spatially and temporally, the acoustic (blast wave) is de-correlated from each fragment due to the slow speed of sound compared to both the bolide speed (~ Mach 60 @ 20 km/s) and compared to the speed of light of the optical pulse (~ one million times faster than the acoustic blast).
Consider the energy of the extreme case of a 1 km impactor compared to the energy of sunlight in one hour on the Earth.
A 1 km bolide with density 2.6 g/cc @ 20 km/s has a KE ~ 3×1020 J
Energy of one hour of sunlight on the Earth ~ 5×1020 J
Comparable but we worry about the 1 km impact, as we should, but not about one hour of sunlight. Yet they have about the same energy on the Earth. Why? Because of the time scale of energy deposition (the power). What PI does is spread the damage from the bolide in both space and time similar to sunlight as an analogy. Of course they are different but the analogy is useful.
The figures below show the overall acoustic, optical and dust production as well as the de-correlated blast waves seen at two ground observers.
Impact threats
The threat of impacts is real and well recorded. Looking at the Moon you can see the craters formed by impacts on the Moon largely frozen in time due to the lack of a lunar atmosphere and hence the lack of weathering and no significant large scale geological upwelling. The Earth has been similarly bombarded but with a few exceptions the evidence is not as immediately visible due to weathering and geological activity as well as most impacts occur in the oceans as the surface of the Earth is largely covered in water.
The time between impacts is reasonably modeled by a power law of time between impacts and the diameter and impact energy of the threat, From geological records we know there have been a number of mass extinction of life on Earth that appear to be associated with impacts from asteroids and comets. The last mass extinction event was about 65 million years ago at the K-T boundary when the dinosaurs were wiped out. In the last century we have witnessed two major events and hundreds of smaller events. Every day approximately 100 tons of small debris rains down on the Earth, burning up in the atmosphere with little effect on us but sometimes the effects can be catastrophic.
Threat Assessment
Asteroid and comet threats are real but highly episodic in terms of loss of life. As discussed below, the threats from asteroid impacts is well approximated by a power law in terms of frequency of events vs energy deposited. In general only asteroids larger than about 20m diameter (occurring on average about every 60 years with about 0.5 Mt energy – assuming stony composition) cause any significant ground damage and only asteroids larger than about 80m diameter (occurring on average about every 5000 years with about 50 Mt energy – assuming stony composition) actually penetrate through the atmosphere and hit the ground. Truly existential threats that could extinguish humanity have diameters great than about 5 km diameter (occurring on average about every 50 million years with about 10 Tt energy – assuming stony composition). The event that killed the dinosaurs (KT extinction) is estimated (based on the Ir in the KT clay layer) to have been a 10 km diameter asteroid (possibly a small comet though it is unclear how the measured Ir would be in a comet) with an energy of approximately 100 Tt or 15,000 times the total energy of the current total worlds nuclear arsenal. Such events are estimated to occur about every 100 million years.
Comet Threats
Comet threats are also very real and potentially much more devastating than asteroid threats. Comets can have extremely large nuclei and are also much higher speed (in general) than asteroids. Much less attention has been placed on comet and comet fragments than asteroids, partly due to their impact rarity compared to asteroids and partly due to our lack of defense options. See our work on directed energy planetary defense for a discussion of comet defense.
Threat Comparison
It is useful to compare various “common threats” we face in life to extraterrestrial threats from asteroids and comets. For example, on average 1.3 million people per year die in car accidents world wide and 50 million people are injured. If the world human population were static (it is not) then in an average human lifetime of about 80 years, 100 million people will die and 4 billion people will be injured in car accidents. Think about this. Half of the world population will be injured (some minor) in an average human lifetime. Why do we drive then? We accept the rate of death and injury largely because it is relatively constant (NOT episodic) AND we need transportation. Imagine for a moment, instead of an average of 1.3 million dying and 50 million being injured per year, that all 1.3 million dead and all 50 million injured happened in one day and then no deaths nor injuries for the rest of the year. This is more like like the analogy to episodic events like asteroid or comet threats. A much more enlightening case is to imagine that all 100 million dead and all 4 billion injured happened in one day and then none for another 80 years (human lifetime). This is the difference between relatively constant events like car accidents and episodic events like asteroid or comets. In general we would NOT tolerate 100 million dead and 4 billion injured in one day but we do tolerate 100 million dead and 4 billion injured in your lifetime. There is a very different psychological response to these scenarios (average death and injury vs episodic) YET the numbers are same. We summarize some threats below.
Relative threat level per Lifetime
C.R. Chapman & D. Morrison, 1994, Nature 367, 33-40
(roughly all humanity dies every 100 million years) 1010/108 = 100 people/yr =10,000 people per human lifetime
~ 10-6 (one chance per million) of a person dying per lifetime
-
Motor vehicle accident = 1 in 100 (1% chance of dying and 50% chance of being injured)
-
Homicide = 1 in 300
-
Fire = 1 in 800
-
aaaaaaaaaaaaaaaaaaaaaaaaaaaaaaaaaaaaaaaaaaaaaaaaaaaaaaaaaaaaaaaaaaaaaaaaaaaaaa Tunguska 1908 – Air burst from estimated 50-100m diameter asteroid or possibly small or grazing comet. Resulting shock blast blew down millions of trees over several thousand square kilometer area.
Firearms accident = 1 in 2,500
-
Electrocution = 1 in 5,000
-
Passenger aircraft crash = 1 in 20,000
-
Flood = 1 in 30,000
-
Tornado = 1 in 60,000
-
Venomous bite or sting = 1 in 100,000
-
Asteroid/comet impact = ~1 in 200,000+ (but Episodic!)
-
Fireworks accident = 1 in 1 million
-
Food poisoning by botulism = 1 in 3 million
-
Drinking water EPA limit of tricholoethylene = 1 in 10 million
Airburst vs Ground Impacts – For common stony asteroids with diameters less than about 80m the asteroid will break up in the atmosphere and not hit the ground. This is known as an “air burst”. For diameters great than 80m there will be a ground impact which is generally far more devastating locally. Our program fractures the bolides (asteroid or comet) so it never hits the ground intact. Very small fragments may survive at hit the ground but they are generally not a danger as they slow down dramatically due to atmospheric drag.
Tunguska – A large event occurred in 1908 in Russia (Siberia – Tunguska event) where a modest sized asteroid or comet fragment estimated to be between 50 and 100m in diameter with and impact energy of between 3 and 30 Mt (megatons TNT) or like that of a large thermonuclear weapon. This event happened in a very remote area that was extremely sparsely populated with the acoustical shock wave knocking down millions of trees over about a 1000 km2 area and was an “air burst event” that did not hit the ground. Had this event occurred over a major modern city it could have killed millions of people. See: Tunguska event – Wikipedia
Chelyabinsk – A recent event that was extremely well recorded was in 2013 on Feb 15 in Chelyabinsk Russia had a diameter of approximately 20m and a yield of about 0.5 Mt or like that of a modern ICBM deployed strategic thermonuclear weapon. This was an “air burst event” that did not hit the ground.
This can seen on numerous “dash cams” from the early morning event. Approximately 1600 people were injured from the blast wave and the resulting window and building damage. Had the impact angle been steeper (closer to vertical) and had this occurred over a large city it could have threatened 10’s to 100’s of thousands of people. See: Chelyabinsk meteor – Wikipedia
Luck vs Threat – So far humanity has been spared large scale catastrophe as was visiting upon our previous tenants but counting upon being “lucky” is a poor strategy in the longer term.

Approximate power law relationship between impact recurrence times and exo-atmospheric impact KE. Power law fits based on fitting data known and extrapolated impacts from [23],[24].
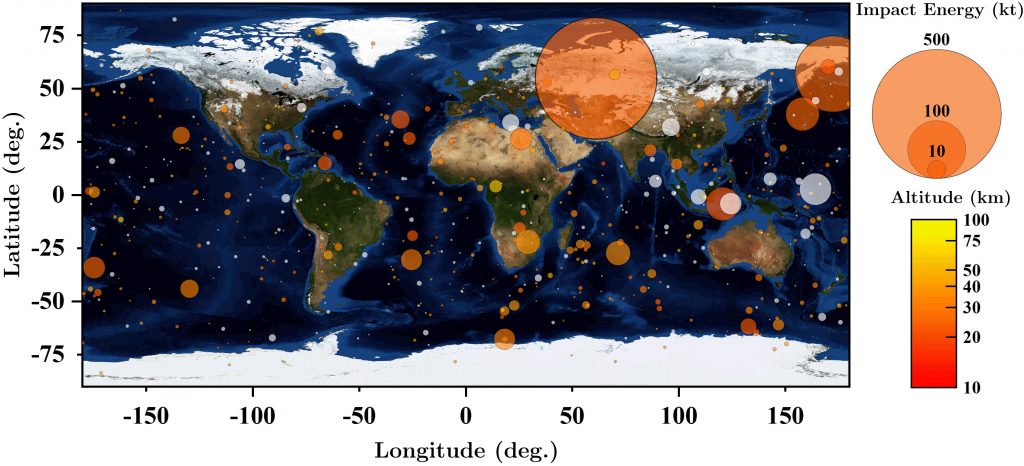
Map of recent 873 events greater than 0.073 Kt from April 15, 1988 to Sept 29, 2021 air burst impacts from atmospheric infrasonic sensors. The Feb 15, 2013 Chelyabinsk 500 Kt event is clearly seen over Russia. It is important to note that the energy ranges of many of these events of asteroid strikes are in the ranges of tactical to strategic nuclear weapons. White colored points lack altitude data. Data from Alan B. Chamberlin (JPL/Caltech) https://cneos.jpl.nasa.gov/fireballs/.
Exo-atmospheric kinetic energy (KE) vs diameter and atmospheric entry speed. Nuclear weapon yields and total human nuclear arsenal shown for comparison.
Intercept Time Sales for various Threats
The PI program is remarkable in the extremely short response times needed for mitigation. These times are vastly less than deflection based ideas for planetary defense. We have done thousands of simulations for various threat scenarios and show some results below for mitigations from 15 to 1000m diameter threats. Below 15m diameter for the most common stony asteroids, no action is needed as the Earth’s atmosphere takes care of the “problem” for us. However, as humanity stretches out into the solar system with lunar and Martian bases with no or lesser atmospheres it is important to implement planetary defense on these outposts as well. In the plot below we show mitigation intercept time scale required for 15, 20, 30, 40, 50, 100, 370 (Apophis), 490 (Bennu) and 1000m diameter threats. We assume a nominal stony asteroid density (2.6 g/cc) and internal binding strength (~1-2 MPa). Note that the impact energy of a threat like Apophis which will visit us next on Friday the 13th of April , 2029 and come closer to the Earth’s surface than geosynchronous satellites, has equivalent energy comparable to the entire nuclear arsenal of the Earth.
Intercept Decision Making
Detection and threat analysis are key to any planetary defense system. Improvements in this area are crucial. Our decision process for a given threat is summarized in the logic flow diagram below with the metric of threat on the surface of the Earth being set by the threshold to keep the blast wave peak pressure low enough to prevent significant damage and the optical pulse kept low enough to prevent fires. While the peak pressure allowed and the peak optical energy flux are free parameters in our simulation we set a limit that is typically <2 KPa for the peak blast pressure and 0.2 MJ/m2 for the sum of all optical pulses. These are shown in the figure above at the bottom for each intercept scenario. These are very conservative assumptions. Every fragment is tracked in our simulations and the atmospheric absorption for both the acoustic and the optical signature is accounted for. A robust planetary “civil defense” notification system is highly desirable to alert people to take shelter and close their eyes and cover their ears.
Robust Defense Capability
A planetary defense system will need both robust detection and a multi layered mitigation capability analogous to national defense systems. In national defense systems there is almost always a terminal defense mode in case the long range mitigation fails or a surprise short time threat occurs. PI offers both a terminal intercept mode for rapid response or as a last line of defense as well as a long range disruption capability if threat detection is early enough, all within the same general “family of mitigation”. Having such a general purpose layered threat mitigation strategy is important to assure compete defense capability as well as reducing the number of mitigation methods for cost effective defense.
Proactive Threat Mitigation
Just as we do in many other areas of life, such as public health in dealing with pandemics proactively by taking protection against many diseases, so too can we treat planetary defense in a proactive way IF we wanted to. While not all threats are “repeat offenders” in that not all threats visit us multiple times before striking, we do know that some threats such as Apophis and Bennu do. One option is to eliminate these threats by preempting them. We could eliminate threats such as Apophis and Bennu with PI by intercepting them on a close approach and eliminating them as a future threat. This is both a policy and a technical area to be discussed.
International Cooperation in Planetary Defense
Ideally the area of planetary defense would transcend national border as the threat respects no borders. An ideal scenario would be to bring together an international effort to defend our planet for future generations.
Conclusion
PI represents a radical new approach to planetary defense. It is practical, testable both in computation, in ground testing of synthetic threats and in orbit. It is a logical and scalable approach that will for the first time allow humanity to have control over its fate.
Blast Wave Simulation Movies
Using the physics discussed in the main paper (http://arxiv.org/abs/2110.07559) we have developed blast wave movies that how the time evolution of the acoustic wave as measured by observers on the Earth’s surface. The pressure is shown vs time and spatial position of the observer We assume the observer are on a flat plane for simplicity. A flat plane is a good approximation over several hundred kilometers. We will continue to add additional simulations as we develop them. Note that you are “seeing” the moving phase front on the blast wave which moves at the projected ground phase speed and not the group speed. Hence the ground phase front speed moves (expands) much faster than the shock speed. The actual shock speed near the ground is slightly larger than Mach 1 while the phase speed can be much faster depending on the projection angle (~ v_shock/sin(theta)) where theta is the angle of any observer point relative to the (particular) fragment burst altitude (typ ~ 30 km). See our paper for the mathematical details of the PI program. Excellent if you are having trouble sleeping.
See our Group YouTube channel for numerous shock wave and optical pulse movies and additional materials:
www.youtube.com/@UCSBDeepspace
Movie below is for 1 day prior to impact intercept of 100m diameter parent asteroid fragmented into 1000 pieces with mean diameters of 10m
This is a near worst case scenario but shows the power of the PI system to mitigate threats
Impact energy is equivalent to approximately 100 Mt (megatons of TNT equivalent) or that of 250-500 thermonuclear weapons on modern ICBM’s
If such an asteroid were to hit a major city it could kill and injure millions of people
Mitigated by PI , what would have been a major catastrophe is reduced to a large light and sound show with some possible limited residential window damage but no direct loss of life nor significant damage
Parent density 2.6 g/cc, 20 km/s speed, 1m/s disruption
Ground grid is 200×200 km at 100m resolution (2000×2000 array) with the center at the spot where the parent asteroid would have hit
Fragment cloud has a mean radius of approximately 86 km
The clock time is the time after the first fragment bursts at about 30 km. The first shock wave hits the ground at t~77 sec
Case Studies
20m diameter
30m diameter
35m diameter
40m diameter
50m diameter
60m diameter
70m diameter
80m diameter
90m diameter
100m diameter
200m diameter
350m diameter – Apophis
500m diameter – Bennu
800m diameter – PDC 2023
1000m diameter
Student Research Team
We thank the many Physics and other department students who have worked on this project. Upcoming papers will feature their work as authors.
Jeeya Khetia (Physics)
Teagan Costa (Physics – graduated 2021)
Hannah Shabtian (Geophysics with Astronomy and Planetary Sciences minor)
Alexander Korn (CCS Physics with Music minor)
Dharv Patel (Physics with Astronomy minor )
Alok Thakrar (DPHS)
Marlon Munoz (Physics)
Kellan Colburn (Physics – graduated 2021)
Albert Ho (Physics)
Brittany Bailey (Geophysics and Planetary Sciences)
Bella Welch (Physics)
Danny Brey (Physics)
Kavish Kondap (Francis Parker HS)
Physics Research Team
Sasha Cohen – Univ of California, Santa Barbara
Jasper Webb – Univ of California, Santa Barbara
Jon Suen – Univ of California, Santa Barbara
Mark Boslough – Univ New Mexico and LANL
Darrel Robertson – NASA Ames
Angela Stickle – Applied Physics Lab – Johns Hopkins Univ
Sasha Egan – New Mexico Institute of Mining and Technology
Elizabeth Silber – Sandia National Lab
Irina Sagert – Los Alamos National Lab – LANL
Oleg Korobkin – Los Alamos National Lab – LANL
Glenn Sjoden – Univ of Utah
Richard Garwin – IBM (Emeritus)
Philip Lubin – Univ of California, Santa Barbara
Related PI References
Lubin, P. “PI – Terminal Planetary Defense”, ASR, 2023
https://arxiv.org/abs/2110.07559
Lubin, P. and Cohen, A., “Asteroid Interception and Disassembly for Terminal Planetary Defense”, ASR, 2023
https://www.sciencedirect.com/science/article/pii/S0273117722009395
Lubin, P. and Cohen, A, “Planetary Defense is Good – but is Planetary Offense Better?”, Scientific American, October, 2021
https://www.scientificamerican.com/article/planetary-defense-is-good-but-is-planetary-offense-better
Lubin, P. et al “PI – Planetary Defense Program Overview”, PDC 2023 – submitted to Acta Astronautica, 2023
Cohen, A., P. et al ” Asteroid Disruption ALE3D Simulations for Planetary Defense via the PI Method.”, PDC 2023 – submitted to Acta Astronautica, 2023
Bailey, B. et al “Acoustical Ground Effects Simulations from Bolide Disruption via the PI Method”, PDC 2023 – submitted to Acta Astronautica, 2023
Patel, D. et al “Optical Ground Effects Simulations from Bolide Disruption via the PI Method”, PDC 2023 – submitted to Acta Astronautica, 2023
Bailey B. et al , “2023 NT1 – A Cautionary Tale” – submitted to Ap J Letters 2023
Funding
Funding for this program comes from NASA NIAC Phase I grant 80NSSC22K0764, NASA NIAC Phase II 80NSSC23K0966, NASA California Space Grant NNX10AT93H as well as from the Emmett and Gladys W. Fund. We gratefully acknowledge support from the NASA Ames High End Computing (HEC) group and Lawrence Livermore National Laboratory (LLNL) for the use of their ALE3D simulation tools used for modeling the hypervelocity penetrator impacts and for funding from NVIDIA for an Academic Hardware Grant for a high end GPU greatly speeding up ground effect simulations.
.